Share this
Organizers:
Buttenschoen & Fletcher
Steffen Rulands
Max Planck Institute"Setting up the epigenome: a collective phenomenon"
Alessandra Bonfanti
University of Cambridge"Characterising the rheology of soft tissues using Fractional Viscoelastic models"
Mathias Sonja
Uppsala University"Impact of force function formulations on the numerical simulation of center-based models"
Clinton Durney
UBC"Quantifying cellular contributions to salivary gland tubulogenesis"
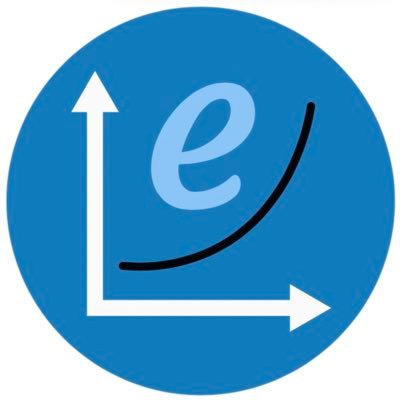