Organizers:
Philip Pearce, Mohit Dalwadi, Alys Clark, Igor Chernyavsky
Description:
Within complex biological systems, transport of nutrients, wastes, cells and signalling molecules is influenced by various physical mechanisms, including fluid flow, diffusion and active transport. To un- derstand the general relationships between structure and function in such systems, it is important to characterise the relative importance to transport of the dominant physical processes, as well as the sys- tem geometry. In recent work, experiments and theory have been used to delineate the effects of geometry, fluid flow, diffusion and active biological processes on transport in various idealised and realistic model systems; in growing systems, the geometry may be coupled to physical processes over certain timescales. This minisymposium brings together experimental and theoretical researchers to investigate the re- lationship between physics and geometry in several complex biological systems. In Part I we focus on bacterial biofilms, in which fluid flow is an important driver of nutrient availability, signalling via quorum sensing molecules, cell erosion, and growth-induced architecture. In Part II we focus on vascular networks and complex tissues, in which fluid flow is the primary mechanism for transport of red blood cells, oxygen and other solutes. The goal will be to discuss the state-of-the-art techniques used to characterise these different systems, including how the associated physical and biological processes interact across different spatial scales, and how to accurately parameterise models across scales. Such techniques are expected to be highly applicable between the systems. Therefore the minisymposium will promote collaboration and help advance research into the broad topic of flow and transport in biology. The minisymposium is intended for those studying specific or general complex biological systems, at any stage in their research careers. The talks will also be useful for any researcher who would like a broad introduction to the applications of fluid mechanics and transport phenomena in biology.
Mohit Dalwadi
Oxford"Using homogenization to determine the effective nutrient uptake in a biofilm from microscale bacterial properties"
Alexandre Persat
EFPL"Cellular advective-diffusion drives the emergence of bacterial surface colonization patterns"
Sara Jabbari
Birmingham"Targeting bacterial adhesion as a novel therapeutic for Pseudomonas aeruginosa infections"
Philip Pearce
Harvard"The biofilm life cycle in high flow environments"
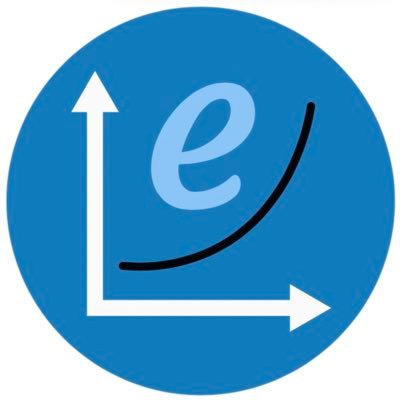